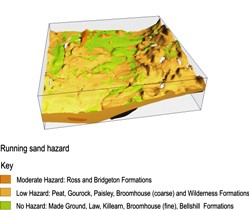
“The ‘Underground Asset Management tool’, under development now, will assist in the maintenance of pipelines, ducts, cables, building foundations, tunnels and any other ferrous materials at risk from attack. Information is provided to aid initial ground investigations and structural design; it also produces a retrospective assessment, and helps managers to prioritise their work on existing structures and pipelines accurately. Eventually the tool will consist of a set of GIS layers for the whole of Great Britain, which, when combined, will provide a corrosivity value, based on information on sulphate and chlorate vulnerability, mineral corrosivity, bedrock and drift geology, shrink-swell, permeability, groundwater levels, and electrical resistivity.”
In future, the hazard susceptibility map idea will be taken to the next stage, by investigating (in collaboration with universities, research institutes and industry partners) various ways of recording national vulnerability to geological hazards. According to Royse, the first phase will look at work carried out by other research councils and academic institutions.
“Eventually we will be able to produce maps of the potential for each geological hazard, with a forward look to the next 10 to 50 years, for the whole of the UK” she says. “Work will also focus on the relationship between climate change and hazard susceptibility.”
LWEC
Industrialists, regulators and decision makers need geo-environmental information to solve the problems created by a growing population, depletion in natural resources, and in response to changes in climate and land-use. For this to happen, BGS will need to provide geoscience data and information that will enable them to understand, as never before, how the Earth system works, how human development is changing it, and how to predict and manage the impacts on the planet, its ecosystems and people.
As Royse says: “Delivering relevant geoscientific knowledge of our dynamic Earth in an accessible way is central to the new BGS Strategy, unveiled at the Royal Society earlier this year. By doing what we do, it is our intention in the Derived Products team to help our wider society make the right choices, in order that it can live with environmental change.”