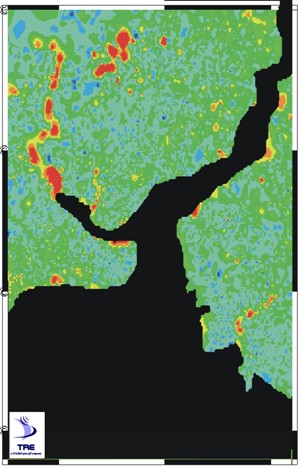
The PSI study of eastern Istanbul shows only very local subsidence, but picks out ancient riverbeds and coastal fills (Figure 7). Ancient riverbeds are abundant because the region has experienced sequences of rapid uplift and inundations during the recent geological past, leaving behind deep, narrow gorges filled with coarse gravel and sand. These riverbeds are barely reflected in actual topography, and in most cases are completely hidden below the modern city.
Conclusions
The GMES Terrafirma project is yielding examples from across the European-Mediterranean region, which show the dramatic contribution that PSI can make to understanding ground movements that threaten urban communities. The potential for extensive mapping and follow-up monitoring, over wide areas at low cost, is a breakthrough which can make a difference to reducing risk through planning and mitigation measures, when coupled with surface-based geological and engineering expertise, and data.
Acknowledgements
The authors would like to thank the Terrafirma Project Core Team, led by Nigel Press Associates (NPA), Tele-Rilevamento Europa (TRE) for the PS processing, and the European Space Agency (ESA) for its sponsorship of the project, and its radar satellite data. BGS contributions are published with the permission of the Executive Director of the British Geological Survey. The European Federation of Geologists (EFG) and EuroGeoSurveys (EGS) are also partners in the project.
References
- Ferretti, A, Prati, C, and Rocca, F, 2001 Permanent scatterers in SAR interferometry IEEE Transactions on Geoscience and Remote Sensing, v 39, p 8-20, doi:101109/36898661
- Donnelly, LJ & Rees, JG 2001 Tectonic and mining induced fault reactivation around Barlaston on the Midlands Microcraton, North Staffordshire, UK Quarterly Journal of Engineering Geology and Hydrogeology, 34, 195-214
- Culshaw, M, Tragheim , D, Bateson, L, & Donnelly, L, 2006 Measurement of ground movements in Stoke-on-Trent (UK) using radar interferometry IAEG 2006 , paper number 125
Visit:
www.terrafirma.eu.com
1 Edinburgh University, West Mains Road, Edinburgh, UK,
[email protected] 2 British Geological Survey, West Mains Road, Edinburgh, UK,
[email protected] 3 University of Florence, Florence, Italy,
[email protected] 4 Geological Survey of Belgium, Brussels, Belgium,
[email protected] 5 British Geological Survey, Keyworth, Nottingham, UK.
[email protected] 6 Kandilli Observatory, KOERI, Istanbul, Turkey,
[email protected]